Division of labour in microorganisms: an evolutionary perspective
The division of labour, whereby individuals within a group specialize in certain tasks, has long been appreciated as central to the evolution of complex biological societies. In recent years, several examples of division of labour in microorganisms have arisen, which suggests that this strategy may also be important in microbial species. In this Opinion article, we explore the set of conditions that define division of labour and propose that cooperation between different phenotypes is a defining feature of division of labour. Furthermore, we discuss how clarifying what constitutes division of labour highlights key evolutionary questions, including what form division of labour takes and why it is favoured by natural selection.
This is a preview of subscription content, access via your institution
Access options
Subscribe to this journal
Receive 12 print issues and online access
206,07 € per year
only 17,17 € per issue
Buy this article
- Purchase on SpringerLink
- Instant access to full article PDF
Prices may be subject to local taxes which are calculated during checkout
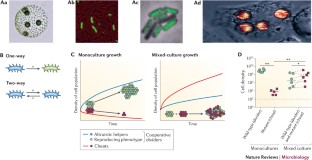
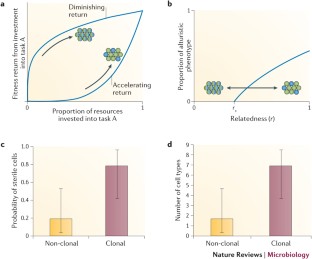
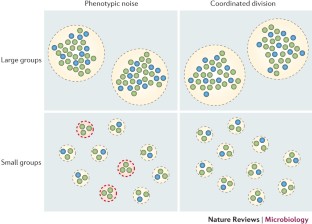
Similar content being viewed by others
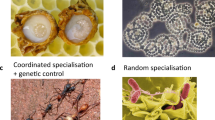
The evolution of mechanisms to produce phenotypic heterogeneity in microorganisms
Article Open access 25 January 2022

Resource sharing is sufficient for the emergence of division of labour
Article Open access 24 November 2022
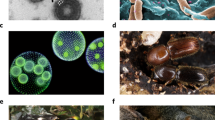
Ten recent insights for our understanding of cooperation
Article 28 January 2021
References
- Lewis, K. Persister cells, dormancy and infectious disease. Nat. Rev. Microbiol.5, 48–56 (2006). PubMedGoogle Scholar
- Veening, J.-W., Smits, W. K. & Kuipers, O. P. Bistability, epigenetics, and bet-hedging in bacteria. Annu. Rev. Microbiol.62, 193–210 (2008). CASPubMedGoogle Scholar
- Ackermann, M. A functional perspective on phenotypic heterogeneity in microorganisms. Nat. Rev. Microbiol.13, 497–508 (2015). CASPubMedGoogle Scholar
- Balaban, N. Q., Merrin, J., Chait, R., Kowalik, L. & Leibler, S. Bacterial persistence as a phenotypic switch. Science305, 1622–1625 (2004). CASPubMedGoogle Scholar
- Veening, J.-W. et al. Transient heterogeneity in extracellular protease production by Bacillus subtilis. Mol. Syst. Biol.4, 1–15 (2008). Google Scholar
- Claessen, D., Rozen, D. E., Kuipers, O. P., Søgaard-Andersen, L. & van Wezel, G. P. Bacterial solutions to multicellularity: a tale of biofilms, filaments and fruiting bodies. Nat. Rev. Microbiol.12, 115–124 (2014). CASPubMedGoogle Scholar
- van Gestel, J., Vlamakis, H. & Kolter, R. in Microbial Biofilms 2nd edn (eds Ghannoum, M., Parsek, M., Whiteley, M. & Mukherjee, P.) 67–97 (ASM press, 2015). Google Scholar
- Ghoul, M., Griffin, A. S. & West, S. A. Toward an evolutionary definition of cheating. Evolution68, 318–331 (2014). PubMedGoogle Scholar
- Voelz, K. et al. 'Division of labour' in response to host oxidative burst drives a fatal Cryptococcus gattii outbreak. Nat. Commun.5, 5194 (2014). CASPubMedPubMed CentralGoogle Scholar
- Maynard Smith, J. & Szathmáry, E. The Major Transitions in Evolution (Oxford Univ. Press, 1998). Google Scholar
- Bourke, A. F. G. Principles of Social Evolution (Oxford Univ. Press, 2011). Google Scholar
- West, S. A., Fisher, R. M., Gardner, A. & Kiers, E. T. Major evolutionary transitions in individuality. Proc. Natl Acad. Sci. USA112, 10112–10119 (2015). CASPubMedGoogle Scholar
- Crespi, B. J. & Yanega, D. The definition of eusociality. Behav. Ecol.6, 109–115 (1995). Google Scholar
- West, S. A., Griffin, A. S. & Gardner, A. Social semantics: altruism, cooperation, mutualism, strong reciprocity and group selection. J. Evol. Biol.20, 415–432 (2007). CASPubMedGoogle Scholar
- Tinbergen, N. On aims and methods of Ethology. Zeitschrift Tierpsychol.20, 410–433 (1963). Google Scholar
- Flores, E. & Herrero, A. Compartmentalized function through cell differentiation in filamentous cyanobacteria. Nat. Rev. Microbiol.8, 39–50 (2009). Google Scholar
- Davidson, C. J. & Surette, M. G. Individuality in bacteria. Annu. Rev. Genet.42, 253–268 (2008). CASPubMedGoogle Scholar
- West, S. A., Griffin, A. S., Gardner, A. & Diggle, S. P. Social evolution theory for microorganisms. Nat. Rev. Microbiol.4, 597–607 (2006). CASPubMedGoogle Scholar
- Hamilton, W. D. The genetical evolution of social behaviour. I. J. Theor. Biol.7, 1–16 (1964). CASPubMedGoogle Scholar
- Grafen, A. Optimization of inclusive fitness. J. Theor. Biol.238, 541–563 (2006). PubMedGoogle Scholar
- West, S. A. & Gardner, A. Adaptation and inclusive fitness review. Curr. Biol.23, R577–R584 (2013). CASPubMedGoogle Scholar
- Ackermann, M. et al. Self-destructive cooperation mediated by phenotypic noise. Nature454, 987–990 (2008). CASPubMedGoogle Scholar
- Diard, M. et al. Stabilization of cooperative virulence by the expression of an avirulent phenotype. Nature494, 353–356 (2013). CASPubMedGoogle Scholar
- Strassmann, J. E., Zhu, Y. & Queller, D. C. Altruism and social cheating in the social amoeba Dictyostelium discoideum. Nature408, 965–967 (2000). CASPubMedGoogle Scholar
- Velicer, G. J., Kroos, L. & Lenski, R. E. Developmental cheating in the social bacterium Myxococcus xanthus. Nature404, 598–601 (2000). CASPubMedGoogle Scholar
- Rainey, P. B. & Travisano, M. Adaptive radiation in a heterogeneous environment. Nature394, 69–72 (1998). CASPubMedGoogle Scholar
- Kim, W., Levy, S. B. & Foster, K. R. Rapid radiation in bacteria leads to a division of labor. Nat. Commun.7, 10508 (2016). CASPubMedPubMed CentralGoogle Scholar
- Griffin, A. S., West, S. A. & Buckling, A. Cooperation and competition in pathogenic bacteria. Nature430, 1024–1027 (2004). CASPubMedGoogle Scholar
- Jiricny, N. et al. Fitness correlates with the extent of cheating in a bacterium. J. Evol. Biol.23, 738–747 (2010). CASPubMedGoogle Scholar
- Andersen, S. B., Marvig, R. L., Molin, S., Krogh Johansen, H. & Griffin, A. S. Long-term social dynamics drive loss of function in pathogenic bacteria. Proc. Natl Acad. Sci. USA112, 10756–10761 (2015). CASPubMedGoogle Scholar
- Diggle, S. P., West, S. A., Griffin, A. S. & Campbell, G. S. Cooperation and conflict in quorum-sensing bacterial populations. Nature450, 411–414 (2007). CASPubMedGoogle Scholar
- West, S. A., Winzer, K., Gardner, A. & Diggle, S. P. Quorum sensing and the confusion about diffusion. Trends Microbiol.20, 586–594 (2012). CASPubMedGoogle Scholar
- Ghoul, M., West, S. A., Diggle, S. P. & Griffin, A. S. An experimental test of whether cheating is context dependent. J. Evol. Biol.27, 551–556 (2014). CASPubMedGoogle Scholar
- van Gestel, J., Vlamakis, H. & Kolter, R. From cell differentiation to cell collectives: Bacillus subtilis uses division of labor to migrate. PLoS Biol.13, e1002141 (2015). PubMedPubMed CentralGoogle Scholar
- Michod, R. E. Evolution of individuality during the transition from unicellular to multicellular life. Proc. Natl Acad. Sci. USA104, 8613–8618 (2007). CASPubMedGoogle Scholar
- Michod, R. E., Viossat, Y., Solari, C. A. & Hurand, M. Life-history evolution and the origin of multicellularity. J. Theor. Biol.239, 257–272 (2006). PubMedGoogle Scholar
- Ispolatov, I., Ackermann, M. & Doebeli, M. Division of labour and the evolution of multicellularity. Proc. Biol. Sci.279, 1768–1776 (2012). PubMedGoogle Scholar
- Gavrilets, S. Rapid transition towards the division of labor via evolution of developmental plasticity. PLoS Comput. Biol.6, e1000805 (2010). PubMedPubMed CentralGoogle Scholar
- Oster, G. F. & Wilson, E. O. Caste and Ecology in the Social Insects (Princeton Univ. Press, 1978). Google Scholar
- Charnov, E. L. The Theory of Sex Allocation (Princeton Univ. Press, 1982). Google Scholar
- Koufopanou, V. & Bell, G. Soma and germ: an experimental approach using Volvox. Proc. R. Soc. B: Biol. Sci.254, 107–113 (1993). Google Scholar
- Rossetti, V. et al. The evolutionary path to terminal differentiation and division of labor in cyanobacteria. J. Theor. Biol.262, 23–34 (2010). PubMedGoogle Scholar
- Wilson, E. O. Ergonomics of caste in social insects. Am. Nat.102, 41–66 (1968). Google Scholar
- Koufopanou, V. The evolution of soma in the Volvocales. Am. Nat.143, 907–931 (1994). Google Scholar
- Herron, M. D., Hackett, J. D., Aylward, F. O. & Michod, R. E. Triassic origin and early radiation of multicellular volvocine algae. Proc. Natl Acad. Sci. USA106, 3254–3258 (2009). CASPubMedGoogle Scholar
- Solari, C. A., Kessler, J. O. & Michod, R. E. A. Hydrodynamics approach to the evolution of multicellularity: flagellar motility and germ–soma differentiation in volvocalean green algae. Am. Nat.167, 537–554 (2006). PubMedGoogle Scholar
- Solari, C. A., Ganguly, S., Kessler, J. O., Michod, R. E. & Goldstein, R. E. Multicellularity and the functional interdependence of motility and molecular transport. Proc. Natl Acad. Sci. USA103, 1353–1358 (2006). CASPubMedGoogle Scholar
- Wolf, J. B. et al. Fitness trade-offs result in the illusion of social success. Curr. Biol.25, 1086–1090 (2015). CASPubMedPubMed CentralGoogle Scholar
- Gilbert, O. M., Foster, K. R., Mehdiabadi, N. J., Strassmann, J. E. & Queller, D. C. High relatedness maintains multicellular cooperation in a social amoeba by controlling cheater mutants. Proc. Natl Acad. Sci. USA104, 8913–8917 (2007). CASPubMedGoogle Scholar
- Mehdiabadi, N. J. et al. Kin preference in a social microbe. Nature442, 881–882 (2006). CASPubMedGoogle Scholar
- Kuzdzal-Fick, J. J., Queller, D. C., Fox, S. A. & Strassmann, J. E. High relatedness is necessary and sufficient to maintain multicellularity in Dictyostelium. Science334, 1548–1551 (2011). CASPubMedGoogle Scholar
- Bastiaans, E., Debets, A. J. M. & Aanen, D. K. Experimental evolution reveals that high relatedness protects multicellular cooperation from cheaters. Nat. Commun.7, 1–10 (2016). Google Scholar
- Fisher, R. M., Cornwallis, C. K. & West, S. A. Group formation, relatedness, and the evolution of multicellularity. Curr. Biol.23, 1120–1125 (2013). CASPubMedGoogle Scholar
- Maynard Smith, J. & Price, G. R. The logic of animal conflict. Nature246, 15–18 (1973). Google Scholar
- Dawkins, R. The Selfish Gene (Oxford Univ. Press, 1976). Google Scholar
- Bonner, J. T. Cellular Slime Molds (Princeton Univ. Press, 1967). Google Scholar
- Shelton, D. E., Desnitskiy, A. G. & Michod, R. E. Distributions of reproductive and somatic cell numbers in diverse Volvox (Chlorophyta) species. Evol. Ecol. Res.14, 707–727 (2012). PubMedPubMed CentralGoogle Scholar
- Bell, G. & Mooers, A. O. Size and complexity among multicellular organisms. Biol. J. Linnean Soc.60, 345–363 (1997). Google Scholar
- Frank, S. A. Host–symbiont conflict over the mixing of symbiotic lineages. Proc. Biol. Sci.263, 339–344 (1996). CASPubMedGoogle Scholar
- Gore, J., Youk, H. & van Oudenaarden, A. Snowdrift game dynamics and facultative cheating in yeast. Nature459, 253–256 (2009). CASPubMedPubMed CentralGoogle Scholar
- Ross-Gillespie, A., Gardner, A., West, S. A. & Griffin, A. S. Frequency dependence and cooperation: theory and a test with bacteria. Am. Nat.170, 331–342 (2007). PubMedGoogle Scholar
- Haig, D. Weismann rules! OK? Epigenetics and the Lamarckian temptation. Biol. Philos.22, 415–428 (2006). Google Scholar
- Maynard Smith, J. Group selection. Q. Rev. Biol.51, 277–283 (1976). Google Scholar
- Gordon, D. M. From division of labor to the collective behavior of social insects. Behav. Ecol. Sociobiol.70, 1101–1108 (2015). PubMedPubMed CentralGoogle Scholar
- Rainey, P. B. & Kerr, B. Cheats as first propagules: a new hypothesis for the evolution of individuality during the transition from single cells to multicellularity. Bioessays32, 872–880 (2010). PubMedGoogle Scholar
- Hammerschmidt, K., Rose, C. J., Kerr, B. & Rainey, P. B. Life cycles, fitness decoupling and the evolution of multicellularity. Nature515, 75–79 (2015). Google Scholar
- Veening, J.-W. et al. Bet-hedging and epigenetic inheritance in bacterial cell development. Proc. Natl Acad. Sci. USA105, 4393–4398 (2008). CASPubMedGoogle Scholar
- Kirk, D. L. Asymmetric division, cell size and germ–soma specification in Volvox. Semin. Dev. Biol.6, 369–379 (1995). Google Scholar
- Schmitt, R. Differentiation of germinal and somatic cells in Volvox carteri. Curr. Opin. Microbiol.6, 608–613 (2003). CASPubMedGoogle Scholar
- Nedelcu, A. M. & Michod, R. E. The evolutionary origin of an altruistic gene. Mol. Biol. Evol.23, 1460–1464 (2006). CASPubMedGoogle Scholar
- Hanschen, E. R., Ferris, P. J. & Michod, R. E. Early evolution of the genetic basis for soma in the Volvocaceae. Evolution68, 2014–2025 (2014). CASPubMedGoogle Scholar
- Fisher, R. A. The Genetical Theory of Natural Selection (Clarendon, 1930). Google Scholar
- Grafen, A. The formal Darwinism project: a mid-term report. J. Evol. Biol.20, 1243–1254 (2007). CASPubMedGoogle Scholar
- Leigh, E. G. When does the good of the group override the advantage of the individual? Proc. Natl Acad. Sci. USA80, 2985–2989 (1983). CASPubMedGoogle Scholar
- Gardner, A. & Grafen, A. Capturing the superorganism: a formal theory of group adaptation. J. Evol. Biol.22, 659–671 (2009). CASPubMedGoogle Scholar
- Shelton, D. E. & Michod, R. E. Philosophical foundations for the hierarchy of life. J. Evol. Biol.25, 391 (2010). Google Scholar
- Sturm, A. et al. The cost of virulence: retarded growth of Salmonella Typhimurium cells expressing type III secretion system 1. PLoS Pathog.7, e1002143 (2011). CASPubMedPubMed CentralGoogle Scholar
Acknowledgements
The authors thank K. Boomsma, A. P. Escudero, K. Foster, A. Gardner, M. Ghoul, J. Gore, A. Griffin, R. May, J. Strassmann, D. Unterweger and J. van Gestel for very useful discussions. The authors also thank M. Ackermann, R. May, R. Michod and J.-W. Veening for kindly providing images. G.A.C was funded by the Engineering and Physical Sciences Research Council (EPSRC).
Author information
Authors and Affiliations
- Department of Zoology, University of Oxford, Oxford, OX1 3PS, UK Stuart A. West & Guy A. Cooper
- Stuart A. West